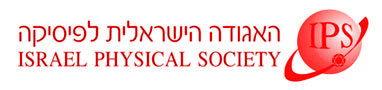
Home
About/Contact
Newsletters
Events/Seminars
2020 IPS Conference
Study Materials
Corporate Members
Home
About/Contact
Newsletters
Events/Seminars
2020 IPS Conference
Study Materials
Corporate Members
Since the early days of IC engines, plasma has played a key role as an ignition method. During the past decade and a half, ever-growing and restrictive demands for increased engine performance and pollutant emission reduction have stressed the need for ignition and combustion processes optimization. As a result the modern IC engine is controlled by an ECU (Engine Control Unit) which processes information supplied by various sensors in order to estimate the desired fuel injection and ignition timing. As part of the same general effort an extensive investigation of homogenous charge compression ignition (HCCI) engines has been conducted all around the world since the late 1990s [1]. It is important to note that the main purpose of an HCCI engine is to enable useful ignition of lean premixed mixtures at relatively high compression ratios. This is achieved by indirect influence of radical generation through chemical kinetic manipulations of the gas mixture, mostly by controlling its chemical composition and temperature. In the past two decades, Plasma Assisted Combustion (PAC) has been widely investigated, mainly in the form of Nanosecond Repetitive Pulse (NRP) discharge. In this process, a voltage of several kilovolts is repeatedly applied for about 10 ns at frequencies of a few tens of kHz. This method has been proven efficient for flame stabilization and reduction of lift-off height [2], expansion of flame extinction limits [3], in cylinder fuel reforming [4] and multiple sites flame initiation [5]. The apparent advantages are all attributed to reaction enhancement by free electron excitation collisions, causing an increase in radical concentration and ultrafast gas heating. In recent years, many fundamental studies of PAC were carried out. These include detailed investigations of the influence of electrical discharges on species concentration and gas temperature [6], identification of involved mechanisms [7], [8] and the influence of NRP in turbulent flow [9]. One major drawback of all of these highly sophisticated methods is the limited ability to measure the state of the intake charge and its chemical advance towards ignition. The measurement methods available today are mostly conducted through several engine working cycles and adjustments are retrospectively made. Our present research was initiated by the idea of using electric breakdown voltage measurements through the gas mixture to evaluate its density. Such measurements can be conducted at high frequencies, potentially even during a single working cycle. The relation between the gas breakdown voltage and its density was already discovered in 1889 by Friedrich Paschen and the basic theoretical foundations were established in 1915 by John Sealy Townsend [10]. In the present research we have conducted breakdown experiments on a conventional automotive sparkplug in nitrogen and generalized Townsends' basic theorem for non – uniform electric fields, produced due to the complex geometry of the sparkplug. At the present stage, we conduct a numerical investigation of the possibility to restrict the NRP method to the streamer discharge regime. This regime can potentially cause volumetric radical generation while maintaining low temperature increases. In addition, we investigate the relation between the change in breakdown voltage between NRP pulses to the advancement towards ignition. If a physically controlled streamer phase restriction is possible, the breakdown voltage between pulses will change with the electrical charge density that exists in the gap. Since these charges are ultimately responsible for radical generation, we suggest that such a method can be applied for both ignition and combustion, optimization and control.
References:
[1] T. K. Sharma, G. A. P. Rao, and K. M. Murthy, “Homogeneous Charge Compression Ignition (HCCI) Engines: A Review,” Arch. Comput. Methods Eng., vol. 23, no. 4, pp. 623–657, Dec. 2016.
[2] D. A. Lacoste, D. A. Xu, J. P. Moeck, and C. O. Laux, “Dynamic response of a weakly turbulent lean-premixed flame to nanosecond repetitively pulsed discharges,” Proc. Combust. Inst., vol. 34, no. 2, pp. 3259–3266, Jan. 2013.
[3] W. Sun, M. Uddi, S. H. Won, T. Ombrello, C. Carter, and Y. Ju, “Kinetic effects of non-equilibrium plasma-assisted methane oxidation on diffusion flame extinction limits,” Combust. Flame, vol. 159, no. 1, pp. 221–229, 2012.
[4] M. Jasiński, M. Dors, and J. Mizeraczyk, “Production of hydrogen via methane reforming using atmospheric pressure microwave plasma,” J. Power Sources, vol. 181, no. 1, pp. 41–45, 2008.
[5] D. Singleton, S. J. Pendleton, and M. A. Gundersen, “The role of non-thermal transient plasma for enhanced flame ignition in C 2 H 4 –air,” J. Phys. Appl. Phys., vol. 44, no. 2, 2011.
[6] J. K. Lefkowitz, P. Guo, A. Rousso, and Y. Ju, “Species and temperature measurements of methane oxidation in a nanosecond repetitively pulsed discharge,” Philos. Trans. R. Soc. Math. Phys. Eng. Sci., vol. 373, no. 2048, 2015.
[7] N. A. Popov, “Fast gas heating in a nitrogen–oxygen discharge plasma: I. Kinetic mechanism,” J. Phys. Appl. Phys., vol. 44, no. 28, 2011.
[8] N. A. Popov, “Investigation of the mechanism for rapid heating of nitrogen and air in gas discharges,” Plasma Phys. Rep., vol. 27, no. 10, pp. 886–896, 2001.
[9] M. Castela, B. Fiorina, A. Coussement, O. Gicquel, N. Darabiha, and C. O. Laux, “Modelling the impact of non-equilibrium discharges on reactive mixtures for simulations of plasma-assisted ignition in turbulent flows,” Combust. Flame, vol. 166, pp. 133–147, 2016.
[10] J. S. Townsend, Electricity in Gases. London: Clarendon Press, 1915.