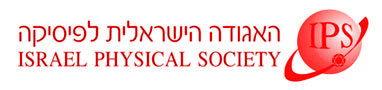
Home
About/Contact
Newsletters
Events/Seminars
2020 IPS Conference
Study Materials
Corporate Members
Home
About/Contact
Newsletters
Events/Seminars
2020 IPS Conference
Study Materials
Corporate Members
Electrons confined to one dimension exhibit various counter-intuitive phenomena such as charge fractionalization, spin-charge separation, and Majorana end modes induced at nanowires rendered topologically superconducting. We study phase coherence length of hot electrons in semiconducting InAs nanowires through scanning tunneling spectroscopic imaging. By maintaining the MBE grown nanowires under ultra-high vacuum we are able to atomically resolve their facets and spectroscopically investigate the quasi-one-dimensional electronic states in them. We visualize the confined nature of these states both through the Van Hove singularities in their spectrum as well as through direct mapping of the subbands via quasi-particle interference. We thus find that relaxation of hot electrons in a multi-channel nanowire is markedly slower for electrons in the lowest subband of the conductance band. Specializing to this channel we identify a new coherent regime of electrons in one-dimension. Above a certain energy threshold the phase coherence length turns non-monotonic where the higher the initial energy of the hot electron is, the more stable it becomes against relaxation. We detect this behavior both in the decay length of the quasi-particle interference patterns at the nanowire end as well as via resonance broadening due to the finite life-time of the hot electrons confined to quantum dots. The latter are formed by a series of stacking faults from which we can extract the high reflectivity of such crystallographic defects. The origin of this unusual energy-evolution of relaxation lies in the form of the Coulomb interaction in one-dimension.